Computational Astrophysics
Theory is how scientists fit data collected from observation into a system of understanding. However, some astrophysical systems are too complicated to be treated adequately using straightforward theoretical calculations. These include the extreme environments near stars, black holes, and other places where strong gravity, magnetic fields, and high-temperature matter combine in complex ways. Systems like those require computational astrophysics, where researchers simulate the system on a computer and compare what they find with observations.
Our Work
Center for Astrophysics | Harvard & Smithsonian astrophysicists use computer models for a huge variety of astronomical systems:
-
Simulating the three-dimensional structure of magnetic fields and materials around a newborn star. The role of magnetic fields in star formation is mysterious, so researchers compare astronomical observations to the results from computational simulations to understand what’s going on.
Chaotically Magnetized Cloud Is No Place to Build a Star, or Is It? -
Modeling spiral galaxies to understand how their spiral arms form. Observations alone can’t tell us how stable spiral arms are over long periods of time, so theoretical astrophysicists use computational models to test plausible explanations for their origin and stability.
New Insights on How Spiral Galaxies Get Their Arms -
Simulating the formation of galaxies on cosmic scales, to model the large-scale structure of the universe. The Illustris Project is a powerful computer program that simulates galaxy formation and merging using models of dark matter and gas particles. This simulation produces a realistic approximation of a part of the cosmic web of galaxies as it evolves over billions of years.
The Cosmic Evolution of Galaxies
The Cosmos Via Computer
Over the past few decades, computers have become fast enough and storage cheap enough to make computational astrophysics a powerful tool for astronomy, alongside telescope or spacecraft observations, theoretical models, and laboratory experiments. Every astronomical researcher today uses computers in some aspect of their work, but computational astrophysics — creating computer models of entire astrophysical systems — is an established method on its own, providing a way to tackle problems that would be otherwise too time-consuming or difficult.
Computational astrophysics is complementary to both lab work and theory, allowing researchers to run many simulations of the same system, or change the parameters of an astrophysical model to check how the outcomes differ. Computation can also be used to compare different models of a system to determine which one fits observational data best.
Computational astrophysics is an important method in many different areas of research:
-
Extreme environments demand computational astrophysics. These include star-forming regions, the environments surrounding neutron stars or black holes, and other places where gravity, magnetism, and high temperatures rule the behavior of matter. Known as magnetohydrodynamics, this research is some of the most computationally challenging but important work in theoretical astrophysics. [ link to “jets shocks & outflows” page ]
-
Galaxies are remarkably complex systems, involving interactions between stars, gas, dust, and the invisible dark matter holding everything together. Astrophysicists studying galactic dynamics model the disks, spiral arms, and other features of galaxies, to compare with observations. Other research simulates galaxy collisions and galaxy formation. [ link to “galaxy formation and evolution” page ]
-
On an even grander level, researchers compute how galaxies form and organize on cosmic scales. These simulations of the large-scale structure of the universe help us understand the “cosmic web” and voids that astronomers see in galaxy surveys.
-
More mundanely, computational astrophysics is necessary when processing massive datasets from telescopes. “Big data” requires computers to process, including new statistical algorithms and machine learning to extract meaning from the sheer amount of raw data produced by modern observatories.
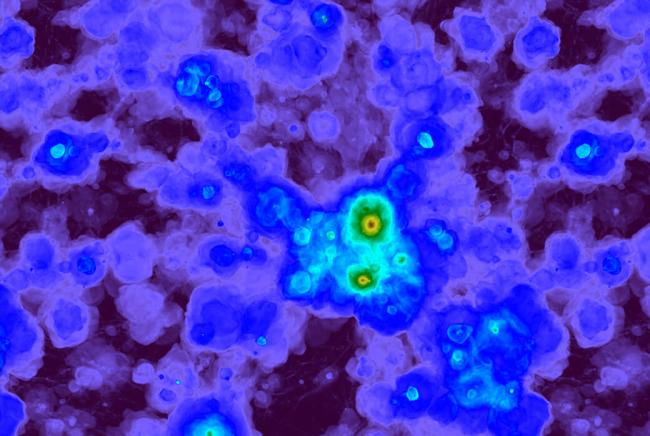
A simulation using the Illustris project of the effect of light from the cosmic microwave background scattering from hot gas in galaxy clusters. This is known as the Sunyaev-Zel'dovich effect, which allows researchers to obtain important information about the evolution of the cosmos.
- How do stars and planets form and evolve?
- What happened in the early universe?
- What do black holes look like?
- Why do galaxies differ so much in size, shape, composition and activity?
- Moons and Satellites
- Neutron Stars and White Dwarfs
- Planet Formation
- Planetary Atmospheres
- Solar and Stellar Atmospheres
- Solar System
- Star Clusters
- Star Formation
- Starburst Galaxies
- Stellar Structure and Evolution
- Supernovas & Remnants
- Variable Stars and Binaries
- Astrostatistics
- Cosmic Microwave Background
- Dark Energy and Dark Matter
- Disks
- Early Universe
- Exoplanets
- Gravitational Dynamics
- Jets, Outflows and Shocks
- Large Scale Structure